Concrete mineralization
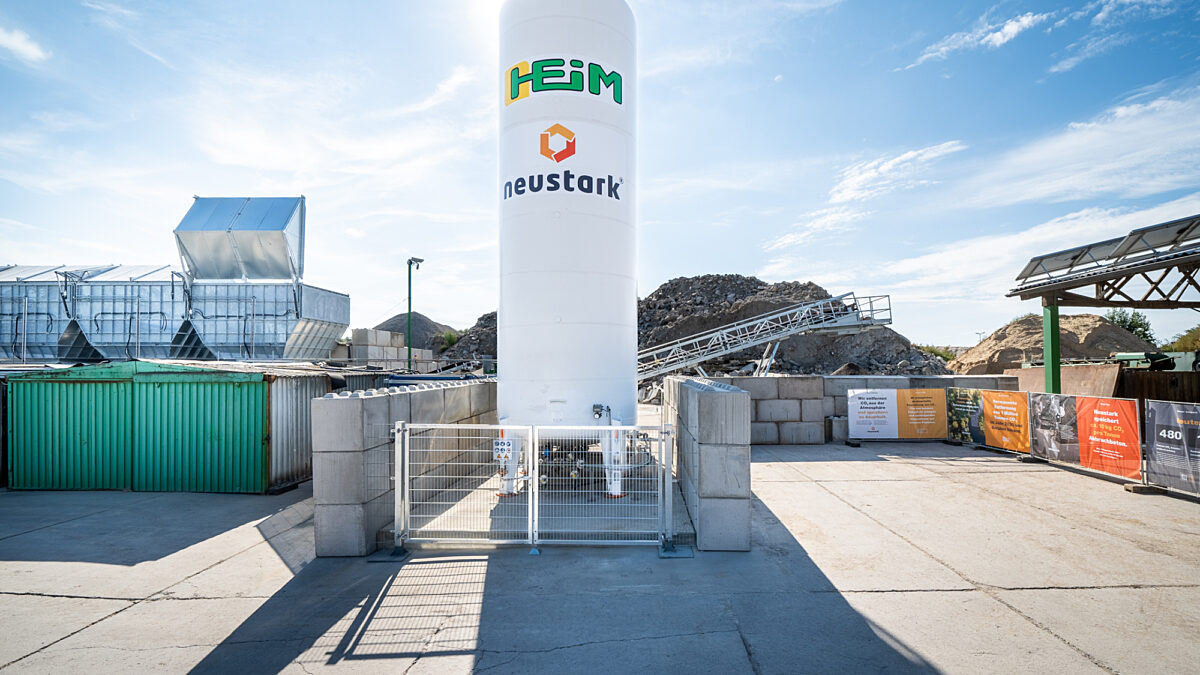

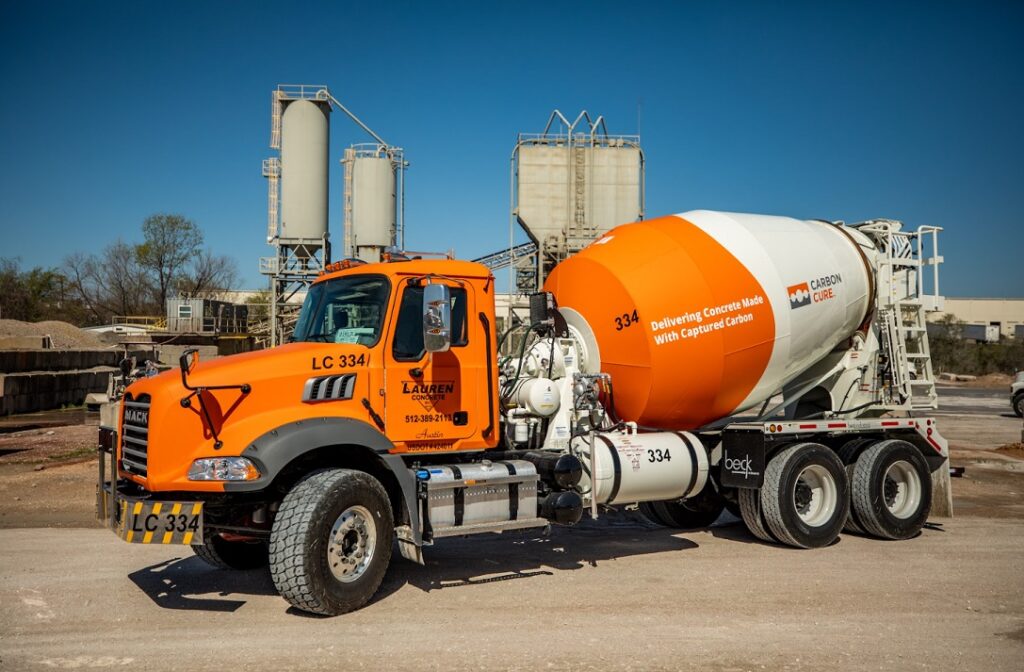
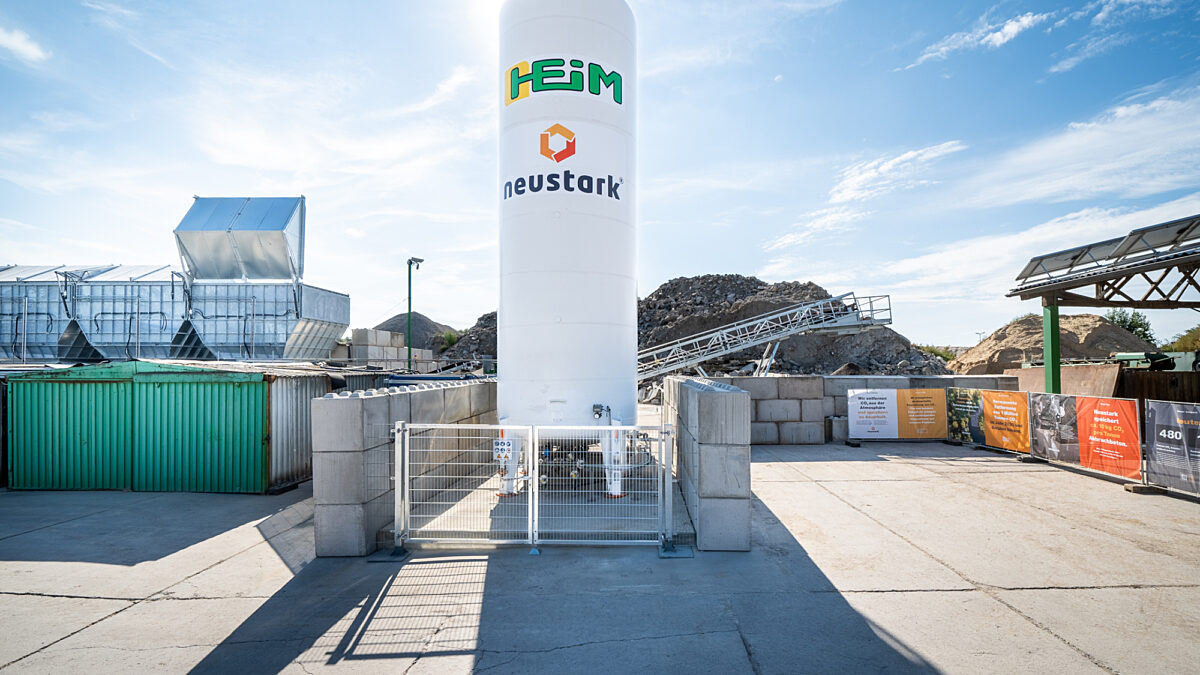

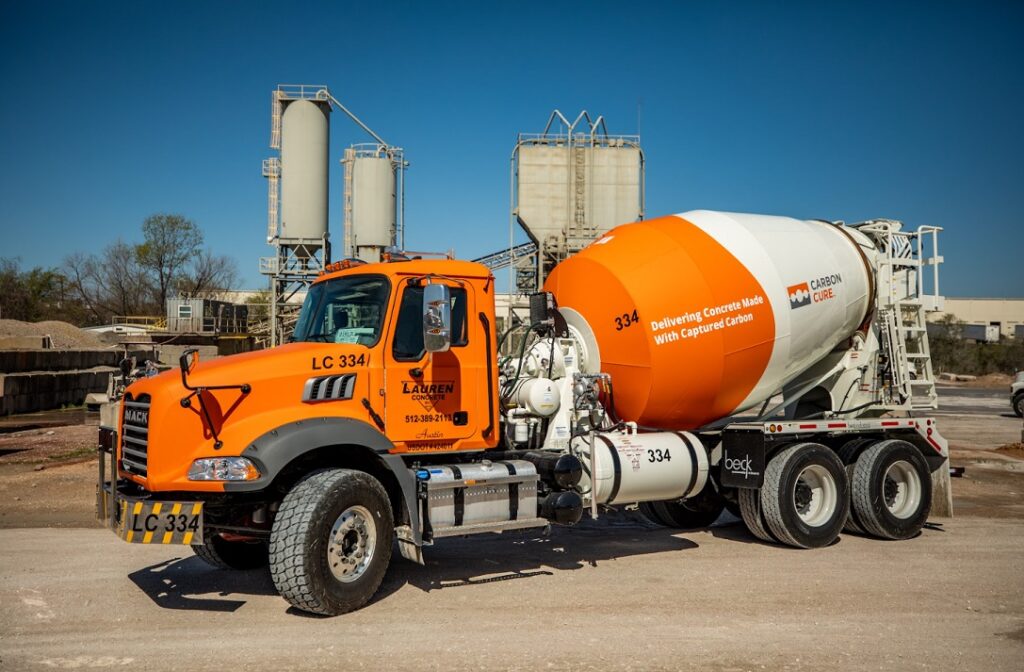
What you need to know about concrete mineralization
- 1Concrete mineralization is the process of adding and storing CO₂ in concrete.
- 2The process could avoid emissions created during the production of cement as well as provide durable CO₂ storage.
- 3The resulting product can be used commercially and may even be stronger than traditional concrete.
- 4Different methods of storage and avoidance are being used and studied, and there is high variability between projects.
- 5Concrete mineralization is often used in conjunction with other projects that use technology to capture CO₂, like direct air capture.
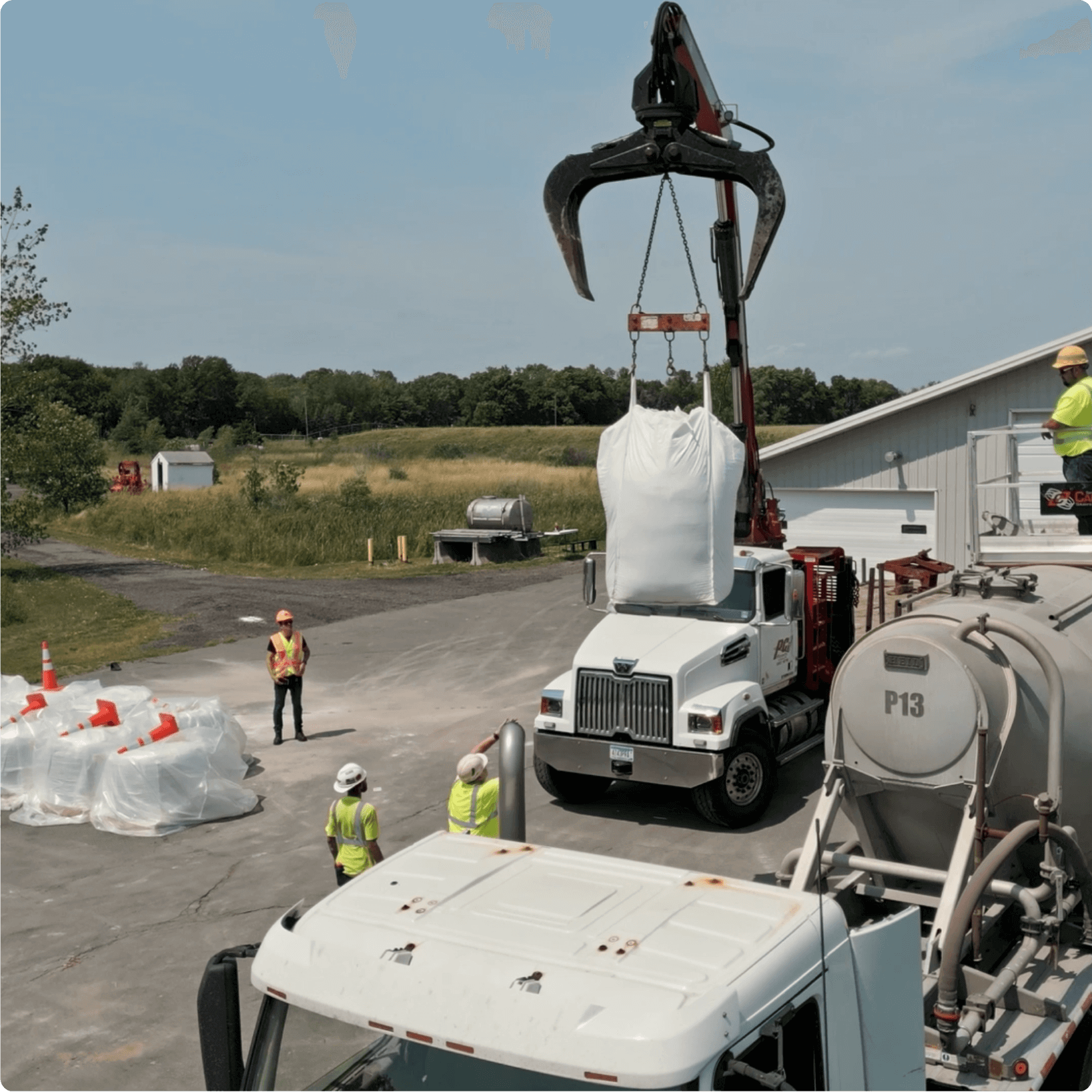
Understanding concrete's role in carbon dioxide removal
Concrete is a mixture of cement (which acts like glue), sand, and crushed rock. While it's essential for building our modern world, concrete production releases a lot of carbon dioxide (CO₂) into the atmosphere. For every tonne of cement made, about a tonne of CO₂ is released. This happens in two ways: burning fuel to heat the kilns, and the chemical reaction that occurs when limestone is processed into cement.
Scientists and engineers are working to transform concrete from being part of the climate problem into part of the solution. While we can reduce fuel emissions by using cleaner energy sources, the CO₂ released during limestone processing is harder to avoid. This challenge has led to new ways of using concrete to capture and store carbon.
What is concrete mineralization?
Concrete mineralization includes several different methods for storing CO₂ within concrete. The first method, called carbonation curing, involves adding CO₂ gas during the concrete mixing or hardening process. This turns certain chemicals in the cement into limestone-like minerals, which can make the concrete stronger and longer-lasting. The process works by injecting captured CO₂ into the concrete mix, which might also reduce how much cement or water is needed.
Another approach adds special mineral ingredients during concrete production. Materials like magnesium oxide and other additives chemically bond with CO₂, locking it inside the concrete. Some versions of this method use waste materials from other industries, while others use new materials. Scientists have tried various improvements, such as adding tiny titanium particles to help concrete store more CO₂, or mixing in biochar (a form of charcoal that is itself a method of carbon removal).
A third method takes advantage of concrete's natural ability to absorb CO₂ from the air over time. This process creates stable minerals within the concrete, similar to how rocks weather naturally. While this happens on its own, scientists have found ways to speed up the process.
Project showcase webinar: Mineralization
In our webinar with Lithos Carbon and CarbonCure Technologies, we explore mineralization via rock weathering and concrete.
The science of carbon storage in concrete
When concrete absorbs CO₂, it undergoes a chemical change. Normally, this natural process (called weathering carbonation) was seen as harmful to concrete because it slowly broke down its structure over time. However, researchers have discovered that when properly controlled, this process can actually make concrete stronger.
Materials that easily bond with CO₂ to form limestone-like minerals often create better concrete, especially in terms of strength and durability. These materials work well because they were previously heated to very high temperatures in industrial processes, making them eager to recombine with CO₂. Steel slag, a waste product from steel manufacturing, is a good example — it readily absorbs CO₂ because it was made from limestone that was heated in steel furnaces.
The history of concrete mineralization
People have been using aggregates to make building materials for thousands of years, and the results of early concrete are still visible in the architecture of the ancient world. Modern concrete — a mixture of cement, sand, and gravel — was invented and put into wide-scale use in the 19th century.
In the early 2000s, scientists and entrepreneurs started wondering what could be done with CO₂ if we needed to avoid it in the atmosphere. The idea started gaining ground as technology advanced, and it gained popularity in the last decade as the importance of decarbonizing the concrete industry became clearer.
Concrete mineralization has grown in popularity alongside other engineered capture methods, like direct air capture (DAC). Establishing storage is essential to DAC, and many air capture projects are using concrete mineralization as their primary form of storage. And, like other engineered capture methods, concrete mineralization is relatively new. More research and rigorous accounting frameworks are vital to the veracity of concrete-based projects.
Concrete mineralization and carbon credits
The carbon credit market offers both immediate and long-term incentives for concrete mineralization projects. Unlike many carbon removal approaches that require years before credits can be issued, certain concrete mineralization methods allow for immediate credit verification based on measured CO₂ injection amounts. This makes concrete mineralization particularly attractive for credit buyers seeking near-term carbon removal confirmations.
However, successful credit projects must carefully consider the concrete's full lifecycle, including its eventual demolition or recycling, as these factors significantly impact the permanence of carbon storage. Carbon credit project developers are increasingly working with construction companies to ensure mineralized concrete can be tracked and properly handled throughout its service life, creating new standards for how carbon removal projects interface with the construction industry.
Measurement, reporting, and verification challenges
Measuring how much CO₂ is stored in concrete requires careful testing and monitoring. The process typically starts at the concrete's surface and moves inward gradually, which can make it tricky to measure accurately since it doesn't always happen evenly.
Scientists can estimate how quickly CO₂ is absorbed using mathematical models that consider factors like concrete strength and environmental conditions. Studies have found that the absorption rate varies widely, and works best when the air humidity is between 40% and 80%.
There are several ways to measure carbon storage in concrete:
First, researchers can track changes in weight as concrete absorbs CO₂. This requires careful measurement and must account for water loss during the process, since the chemical reaction produces heat that makes water evaporate.
Second, they can use special equipment to analyze how the concrete's composition changes as it absorbs CO₂. For small samples that need very precise measurement, scientists use sophisticated laboratory equipment. Larger samples can be tested with simpler methods, though these aren't quite as accurate.
Long-term storage and environmental impact
How long CO₂ stays locked in concrete depends largely on what happens to the concrete over time. Research shows that over 100 years, concrete can naturally absorb between 37% and 86% of the CO₂ that was released to make it. A global study found that concrete structures built between 1930 and 2013 have absorbed about 43% of the CO₂ that was released to make them.
What happens to concrete at the end of its life greatly affects its carbon storage. When old concrete is demolished and crushed into smaller pieces, it actually absorbs CO₂ faster because more surface area is exposed to air. However, if concrete is buried in landfills, it absorbs CO₂ more slowly.
When evaluating concrete's environmental impact, several factors need consideration:
- The CO₂ released during mining and transporting limestone
- Where the CO₂ used in the process comes from
- How much energy is needed to add CO₂ to concrete
- Whether the concrete can be reused or recycled
Practical implementation and industry changes
Using concrete for carbon storage requires significant changes to how the construction industry operates. Concrete production is heavily regulated with strict safety standards, and any changes to traditional methods need extensive testing and approval.
The construction industry tends to be cautious about adopting new techniques, since concrete's reliability is crucial for safety. However, recent concerns about concrete durability have created an opportunity for innovation. While this opens the door for new carbon-storing technologies, changing industry standards and building codes takes time.
Future prospects and scaling up
While storing carbon in concrete shows promise as a large-scale solution for removing CO₂ from the atmosphere, several practical challenges need to be addressed. The technology must prove it can safely store meaningful amounts of CO₂ while meeting all construction safety requirements and building codes.
Making this technology widespread requires considering:
- Modifying existing concrete plants to handle new processes
- Training workers in new techniques
- Setting up systems to measure and verify carbon storage
- Making sure the process remains cost-effective
Concrete mineralization offers an innovative way to fight climate change by using one of our most common building materials to store CO₂. The variety of available methods provides flexibility, though this also creates challenges for standardizing practices and verifying results.
While the basic science is well understood, putting it into practice requires overcoming significant technical and regulatory hurdles. As measurement techniques improve and industry standards adapt, storing carbon in concrete could become an increasingly important tool for removing CO₂ from the atmosphere.
Success depends on finding the right balance between storing CO₂ and maintaining concrete's essential properties as a building material. As the technology develops, projects must prove they can both effectively store carbon and produce reliable concrete that meets the construction industry's high safety standards.
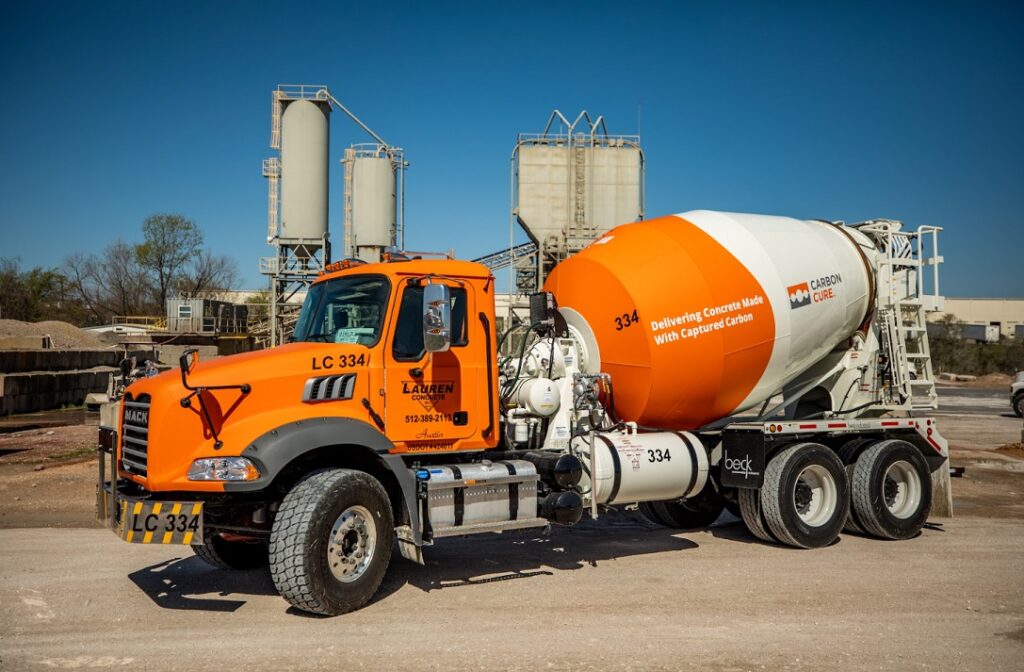
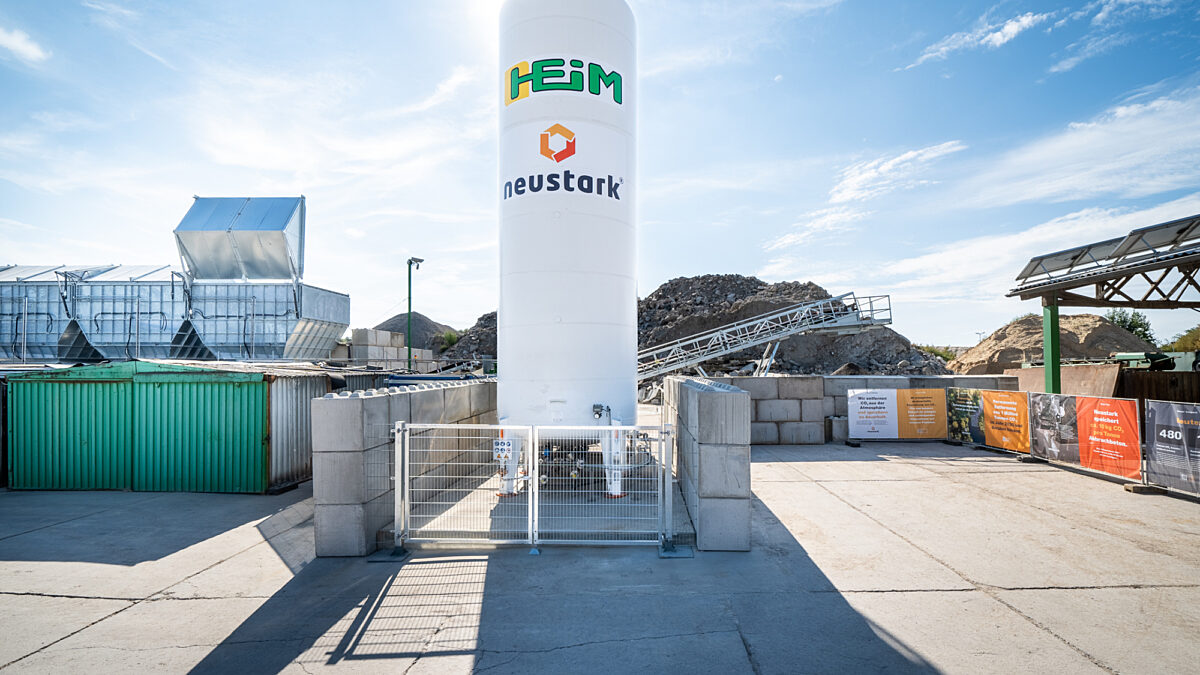
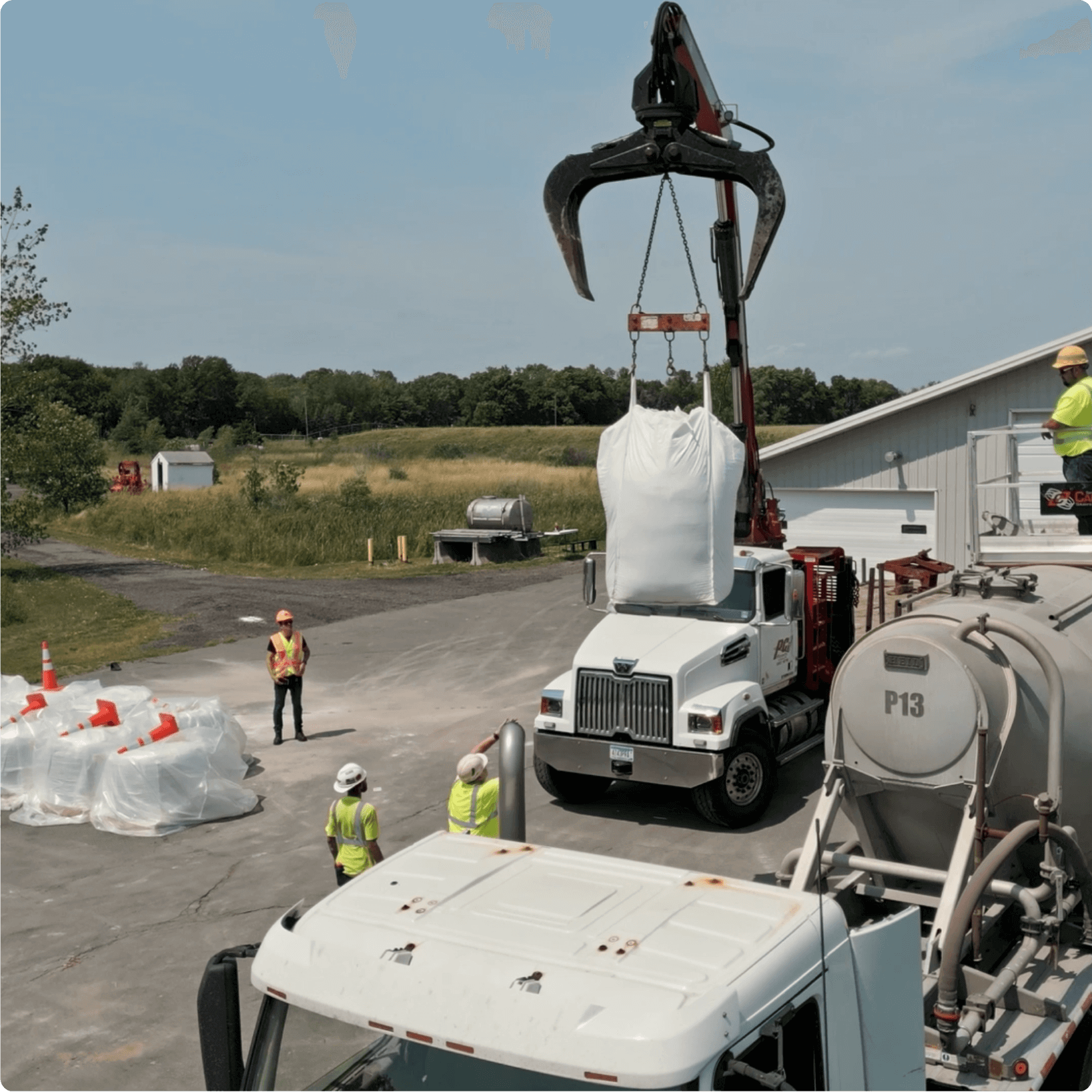
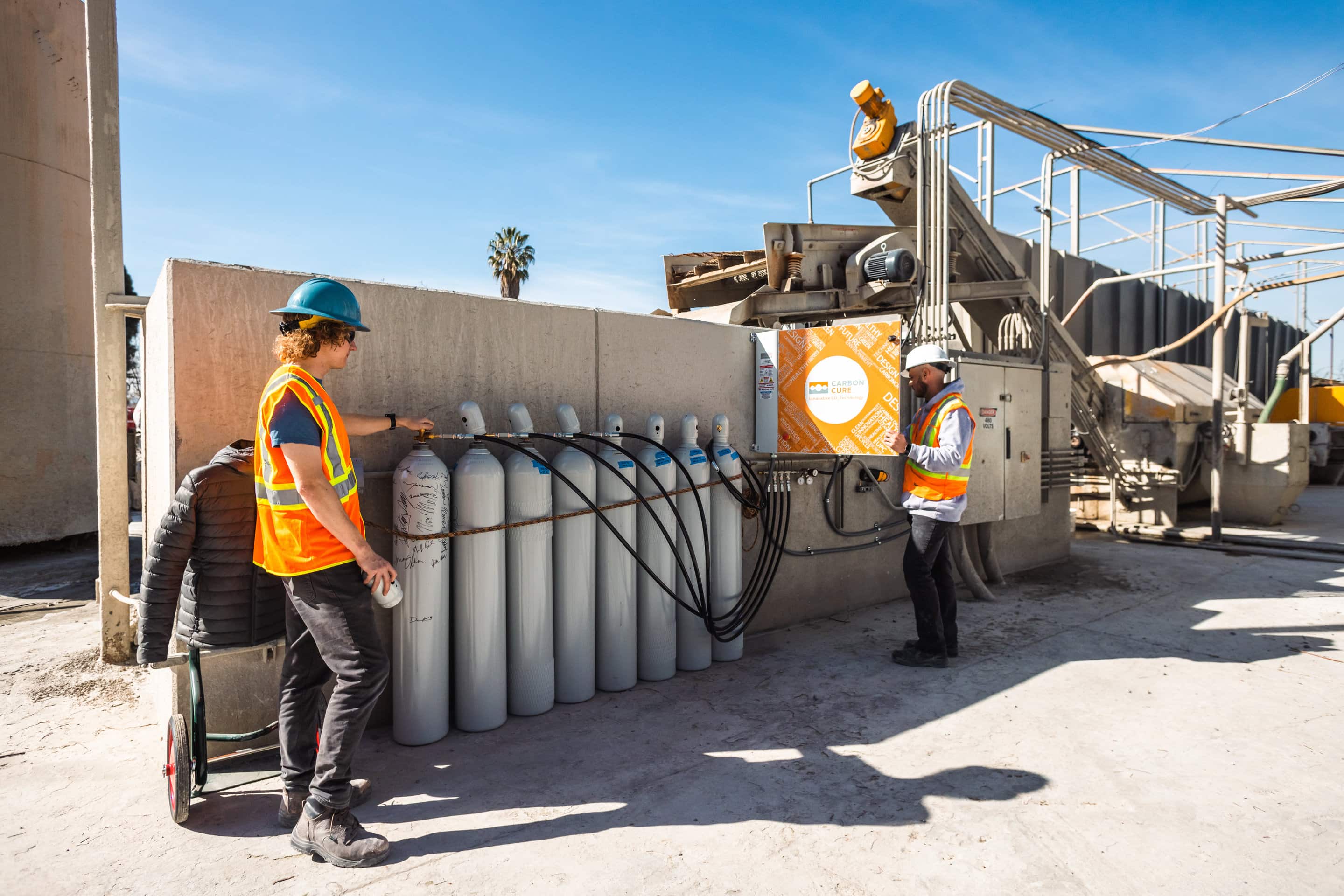
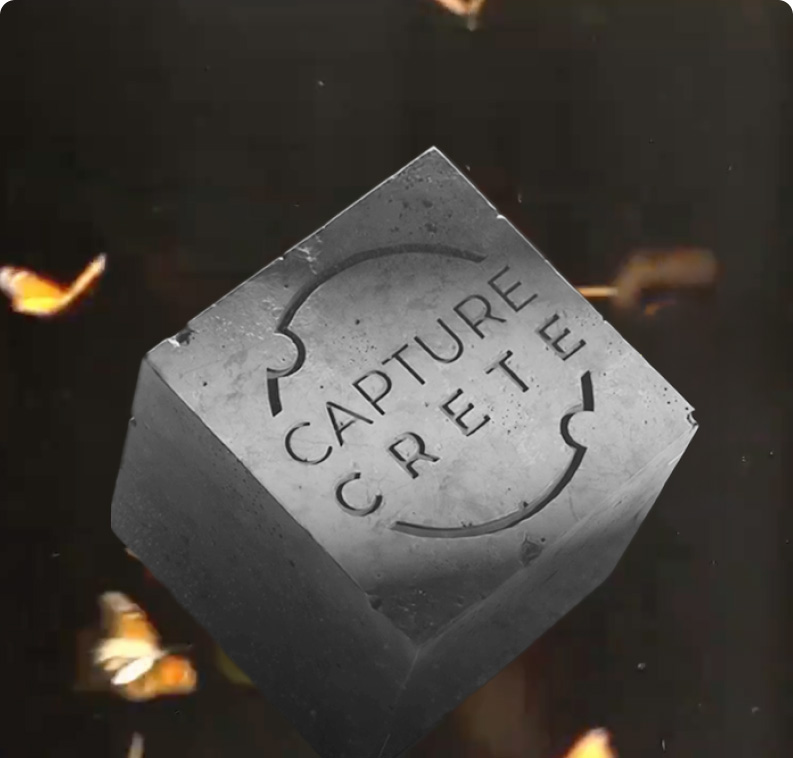
For direct CO₂ injection methods, the amount stored can be measured immediately at the point of injection, allowing for quick verification and credit issuance, unlike many other carbon removal approaches that require years of monitoring.
Once CO₂ is mineralized into concrete, the chemistry ensures minimal leakage risk, with studies showing storage potential of 60+ years in typical construction applications and even longer when concrete is properly recycled.
The massive existing concrete industry infrastructure means mineralization projects could scale rapidly once technologies are proven, with potential implementation at thousands of existing concrete plants worldwide.
Since concrete naturally absorbs 37-86% CO₂ over 100 years, buyers must carefully verify that mineralization projects achieve storage beyond this baseline to ensure credits represent truly additional carbon removal.
Building codes and industry regulations require extensive testing and modification to accept mineralized concrete, which could delay or prevent projects from reaching scale and delivering promised carbon removal.
While initial CO₂ injection can be measured easily, long-term storage verification becomes more complex as concrete ages and moves through different use phases, potentially creating uncertainty about the permanence of carbon storage claimed in credits.
Want to accelerate climate solutions like concrete mineralization? We want to hear from you.
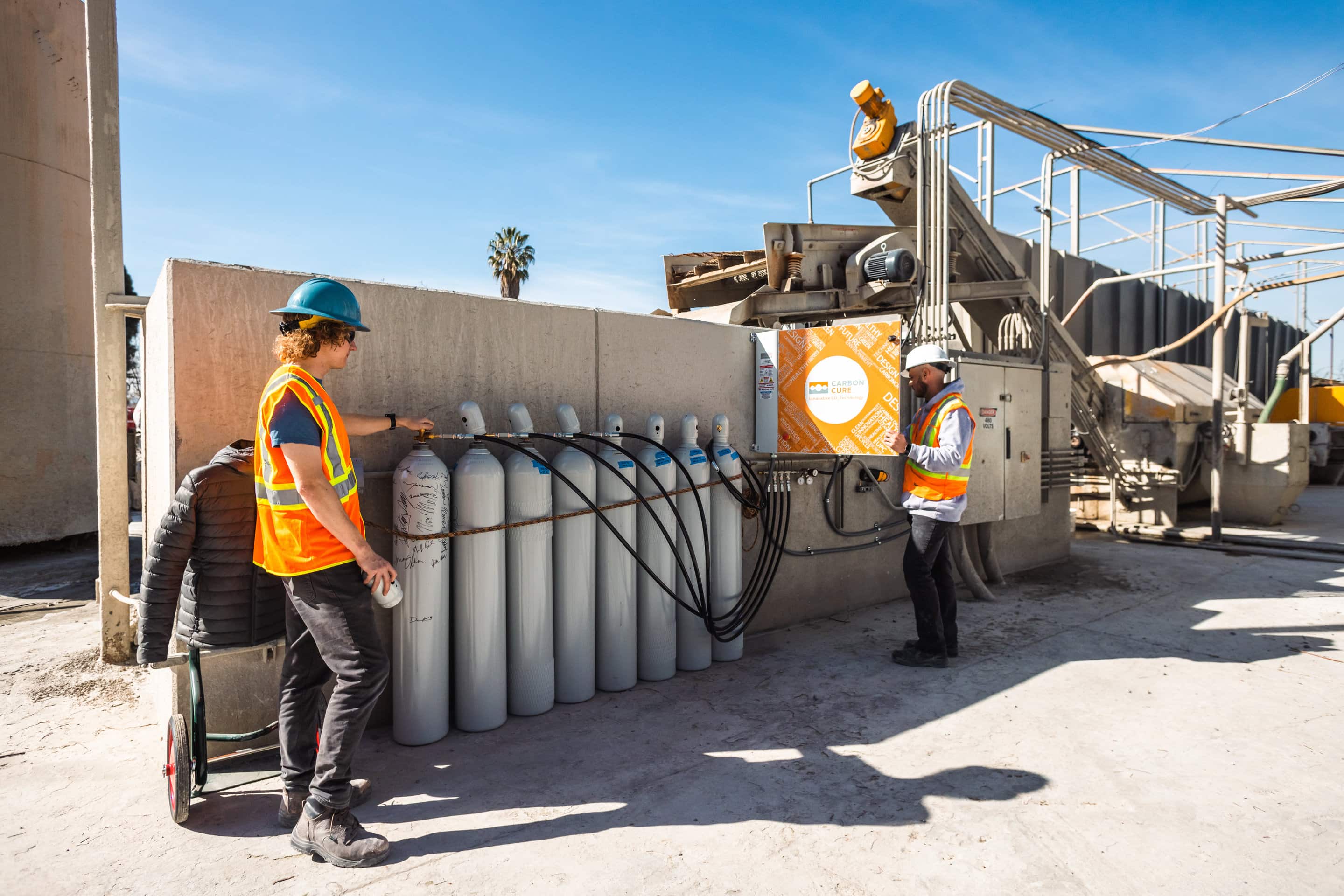